Transport tunnels in enzymes: a novel avenue for protein engineering to improve or modify the catalytic function
Adapted from Tunnels modulate ligand flux in a heme nitric oxide/oxygen binding (H-NOX) domain, PNAS https://doi.org/10.1073/pnas.1114038108
Catalytic proteins or enzymes have fascinated biochemists and chemists alike. The ability of enzymes to perform many seemingly difficult reactions readily is simply astounding. Enzymes are capable of performing highly endergonic chemical reactions at ambient or near ambient temperatures. Enzymes can catalyse intricate chemistries in the highly crowded macromolecular milieu of cytoplasm with astounding fidelity. Enzymes are capable of immense selectivity so that even a less favorable reaction becomes highly preferred one in the presence of an enzyme despite the presence of competing chemical reactions that are thermodynamically more favorable. The amazing selectivity and fidelity of enzymes is an essential requirement for the fundamental molecular processes and metabolism, without which no life-form can exist. The question is how do enzymes achieve this degree of selectivity and fidelity? The answer is in the fine structural details of the proteins. Enzymes achieve this feat of precision, selectivity and fidelity by elements of molecular selectivity present within the structure of proteins.
Certain enzymes have a deeply buried catalytic center in the interior of the protein, this is connected to the surrounding solvent via empty spaces that have catalytic center at one end and open to the surrounding solvent at the other end. Such conduits are known as tunnels, they facilitate the transport of ligand and product molecules to and from the catalytic center. Recent studies have uncovered that molecular tunnels are important determinants of catalytic activity, not only activity but also other key attributes such as substrate specificity and enantioselectivity (favoring one enantiomer over another). Therefore, tunnels are not just the empty spaces or voids in the protein structure, but rather they are dynamic conduits or pathways that mediate transfer of substrates and products to and from the active site of the enzymes, respectively. The functional relevance of their existence is not limited only to basic functions of ligand or product transport but they do other things such as excluding the bulk solvent from the site of catalysis, secluding the highly reactive intermediates inside the protein cavity, controlling the access of ligands to the active site.
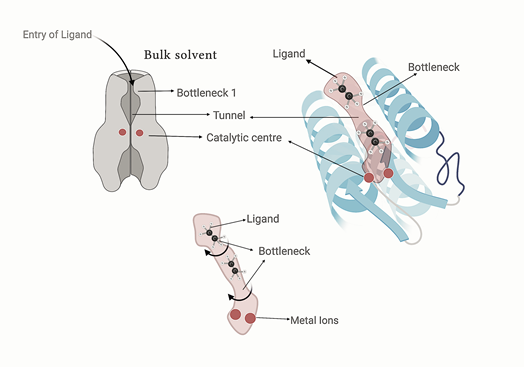
A closer inspection reveals that tunnels are subject to evolutionary pressures and show modifications from one orthologue to another within the same family. Hence changes in the enzyme tunnels or ligand transport pathways can dramatically affect the rate of an enzymatic reaction, specificity or promiscuity for certain substrates, enantioselectivity and even the overall stability of the enzymes.
Up until now, biochemists, enzymologists and protein chemists have focused on the engineering of the catalytic center of the enzyme to alter its catalytic properties. But with emerging data, it is becoming widely accepted that the ligand transport pathways can substantially influence catalytic properties and now they are targeted for protein engineering to modify the catalytic profile of high-value enzymes. There have been significant advances in computational tools available for identification and characterization of molecular ligand transport pathways. Earlier the computational tools to compute the tunnels were good enough to only predict the tunnels in static structures. But now sophisticated tools based on Voronoi diagram algorithms such as CAVER analyst and MOLE are available to predict the pathways in the dynamic protein structures, the input file for these tools is Molecular dynamics simulation of proteins. These tools analyze the dynamic tunnels or ligand transport pathways in the protein structures, compute their relative preponderance, physical parameters such as average bottleneck radius, maximum bottleneck radius, length, curvature and other such parameters along with the list of amino acid residues that surround the inside of the tunnel. Such residues are called tunnel residues, even more important are the ones that are around the narrowest part in the tunnel, known as tunnel bottleneck. Such residues are called bottleneck residues.
These tools can inform a lot about the most probable pathways for ligand ingress and product egress from the enzyme, and by combining the experimental data with these predictions, protein engineers can modify the pathways (for example replacing bulkier amino acids by smaller ones to reduce bottleneck hindrance) or even create alternate pathways or tunnels in the protein structure. However, it is not always beneficial to reduce the bottleneck, sometimes it is more helpful to accentuate an existing bottleneck or to create one de-novo.
For instance, a dehalogenase enzyme was engineered by introducing bulky residues in the transport tunnel, the advantage of doing such mutations was to prevent the water from entering into the active site, which promoted the activated complex formation and thereby enhanced the rate of the catalytic reaction. This allowed the engineered enzyme to acquire a 30-fold increase in the activity against the anthropogenic compound 1,2,3-trichloropopane.
Similarly, as shown in the opening vignette, the tunnel blocking mutations have modulated the heme ligand properties in H-NOX enzyme. By introducing a bulky tryptophan residue in one of the main tunnels leading to the catalytic site of the enzyme, one can effectively trap the substrate near the active site, increasing its local concentration, which in turn increases CO Kon and at same time decreases CO Koff and hence an overall increase in catalytic activity. Hence the engineering of substrate tunnels by mutations requires a deep understanding of underlying catalytic mechanisms and implications of introducing a mutation on the overall catalytic activity of the enzyme.
Selected readings
- Kokkonen P, Bednar D, Pinto G, Prokop Z, Damborsky J. Engineering enzyme access tunnels. Biotechnol Adv. 2019;37(6):107386. doi:10.1016/j.biotechadv.2019.04.008
- Petrek M, Kosinová P, Koca J, Otyepka M. MOLE: a Voronoi diagram-based explorer of molecular channels, pores, and tunnels. Structure. 2007;15(11):1357-1363. DOI:10.1016/j.str.2007.10.007
- Tunnels modulate ligand flux in a heme nitric oxide/oxygen binding (H-NOX) domain, PNAS https://doi.org/10.1073/pnas.1114038108
- Chovancova E, Pavelka A, Benes P, et al. CAVER 3.0: a tool for the analysis of transport pathways in dynamic protein structures. PLoS Comput Biol. 2012;8(10):e1002708. DOI:10.1371/journal.pcbi.1002708
- Pavlova M, Klvana M, Prokop Z, et al. Redesigning dehalogenase access tunnels as a strategy for degrading an anthropogenic substrate. Nat Chem Biol. 2009;5(10):727-733. DOI:10.1038/nchembio.205